Emerging Technologies
Jump to: Offshore Wind | Small Modular Reactors | Enhanced Geothermal Systems | Wave Energy | Carbon Capture and Sequestration | Description of Proxy Emerging Tech Reference Plant
As states and utilities progress toward clean, non-carbon emitting energy portfolios, there are opportunities for new, emerging supply-side technologies to compete with established renewable resources—such as onshore wind and solar photovoltaic—and that will play a critical role in the future power system. The Council defines long-term, emerging technologies as resources and technologies that have a long-term potential in the Pacific Northwest but are not commercially available or deployable on a large scale at the beginning of the power planning period – per the Council’s categorization of new generating resource options. There are several emerging technologies that meet this definition and could play a role in the future Pacific Northwest power system, including offshore wind, small modular nuclear reactors (SMR), enhanced geothermal systems (EGS), ocean energy, and various carbon capture and sequestration technologies.
As part of the research and development initiatives in the 2021 Power Plan, the Council recommends that regional entities work with developers and manufacturers to explore the regional resource potential of supply-side emerging technologies. In addition, the Council urges the region to identify potential barriers to deployment, including costs, transmission, siting, etc., and work together toward solutions when it is in the best interest of the region.
The Council decided to model one emerging technology as a proxy to represent the potential of any emerging technology that could be commercially available in the next ten years. When considering which technology to base the reference plant on, the Council qualitatively analyzed and discussed with the Generating Resources Advisory Committee (GRAC) the following emerging technologies - offshore wind, small modular reactors, enhanced geothermal systems, wave energy, and carbon sequestration technologies. While each of these resource alternatives possess unique attributes that could be beneficial to the future power system, there are also unique challenges remaining - including cost, dependence on technological advancements, licensing, mitigation of environmental effects, waste disposal, and public perception - in order to reach full commercial operation in the region. In addition to the detailed presentations on each of the emerging technologies, brief summaries are provided below.
Offshore wind, while an established resource in other parts of the world, is still emerging in the Pacific Northwest. Offshore wind turbines are similar to their onshore counterparts, except that they tend to be much larger in both size and energy output (a typical onshore wind turbine installed today is about 3 MW, while an offshore wind turbine is closer to 10 MW). There are multiple turbine technologies that can be used, depending on the area and depth of the body of water. In shallow waters, a fixed-bottom turbine can be deployed, where the device is installed and connected to a foundation in the seabed (or floor of another body of water). Once the water depth gets to around 60 meters and greater, a fixed-bottom installation is no longer feasible. Instead, a floating turbine is required – a turbine that sits atop a floating platform that is tethered to the seabed to prevent it from drifting. Floating turbine technologies are less established than fixed-bottom, but they open up significantly more potential for offshore wind in terms of access to more viable wind speed sites.
NREL Depiction of Offshore Wind Technologies
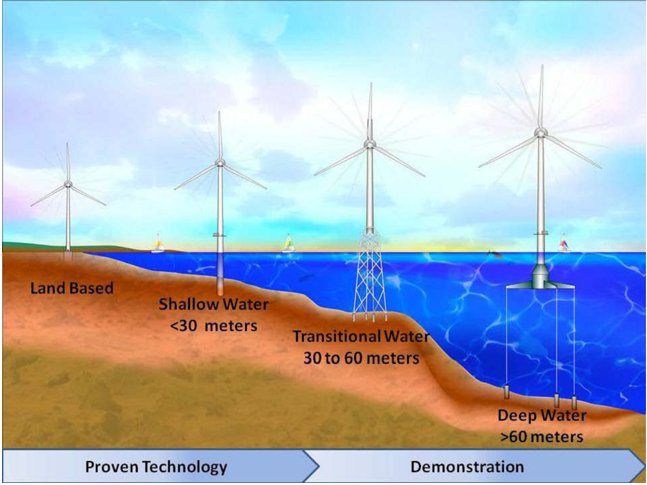
In the Pacific Northwest, while there is tremendous potential for offshore wind, the majority of it (97% of the 62 gigawatts of available technical potential in Oregon, according to a 2019 report by NREL) exists in water depths greater than 60 meters. This will require floating turbines, of which there are varying concepts and designs in the research and development process.
Nuclear power plants produce electricity from energy released by the controlled fission of certain isotopes of heavy elements such as uranium, thorium, and plutonium. Nuclear is a source of dependable capacity and baseload zero-carbon energy that is largely immune to variable fuel prices and usually eligible to meet clean policies. A new conventional nuclear unit would entail the risks of construction delay to an already lengthy construction lead time, escalating costs, and the reliability risk associated with a large single-shaft machine. Rather, the emerging small modular reactor (SMR) technology’s smaller size and modular construction is intended to reduce capital cost and investment risk by utilizing a greater degree of factory assembly, shortening construction lead time, and better matching plant size to customer needs and finances through scaling of multiple units. The smaller plant size of SMRs may also permit greater siting flexibility, load following capability, and cogeneration potential and can benefit system reliability through reduction in “single shaft” outage risk with multiple independent reactor-generator pairings. One of the major topics (and public perception issues) with any nuclear power plant is spent fuel management – responsibly and safely storing and disposing of used fuel – and small modular reactors are no exception.
There are several SMR projects currently being explored in the region – the Utah Associated Municipal Power Systems (UAMPS) Carbon-Free Power Project (CFPP) based on NuScale technology, the Tri-Energy Partnership (X-energy, Grant County PUD, and Energy Northwest) to develop an advance Xe-100 reactor, and the TerraPower Natrium technology (coupling an advance nuclear unit with a molten salt energy storage system) in collaboration with Energy Northwest.
NuScale Small Modular Reactor Design
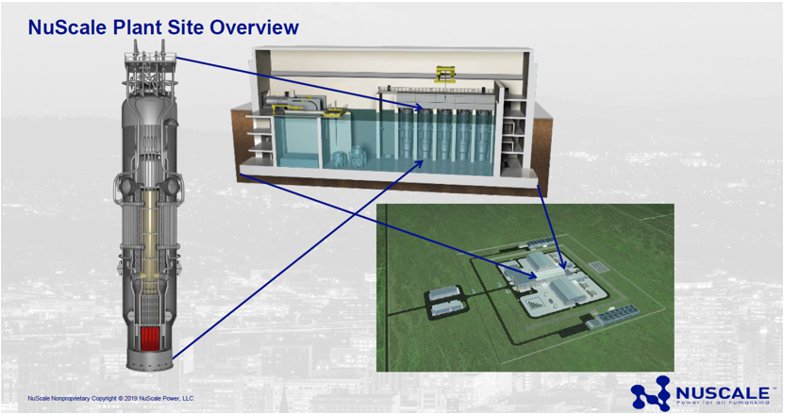
While conventional geothermal requires the natural occurrence of heat, water, and permeability, enhanced geothermal systems (EGS, also known as “engineered” geothermal systems) only require the presence of hot rock. The permeability of the rock and the fluid are engineered through sub-surface fracturing and injection wells. Because hot rock exists everywhere beneath the Earth’s surface (at varying depths), the geographic potential for EGS as a resource is greater than conventional geothermal - which is currently limited to known naturally occurring sites that indicate the presence of geothermal activity (e.g. geysers). See the table in the conventional geothermal reference plant for potential of geothermal and EGS in the region.
In addition to EGS, there is a further emerging technology known as “super hot rock” EGS, which taps into rock 400° Celsius and greater and at depths 5 kilometers and greater (often closer to 20 kilometers) – significantly amplifying the energy potential of the resource. See graphic below from AltaRock, which describes the energy potential per well for natural gas, conventional geothermal, EGS, and the theoretical application of super hot rock EGS. However, in order to tap into super hot rock EGS, technological advances must occur in drilling equipment and techniques to safely reach the depths required. There have been several efforts to explore and drive research of EGS and super hot rock EGS in the Pacific Northwest, including by AltaRock and the Pacific Northwest National Laboratory (PNNL).
Comparison of Energy Potential at Varying Depths and Temperatures
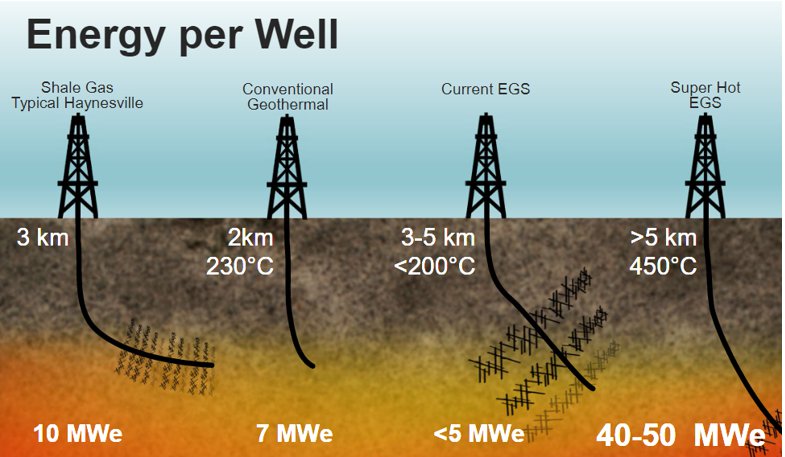
* Image courtesy of AltaRock (see presentation to the GRAC)
Beyond traditional hydroelectric power, there are other energy resources that can be derived from the naturally occurring phenomenon in the Earth’s oceans and rivers and harnessed into electricity, including currents, tides, and waves. While all are considered emerging, wave energy appears to be an appealing match for the Pacific Northwest power system with high energy potential along the Pacific coastline from California to Alaska. Wave power devices and converters capture energy through motion at the surface or through the pressure fluctuations from the waves below the surface. While highly seasonal and subject to storm-driven peaks, wave energy is relatively continuous and predictable.
The US Department of Energy is funding and developing a wave energy test facility off the Oregon Coast called PacWave South. This site is intended for developers to test different wave energy converter devices in a fully operating, 20-megawatt, grid-connected facility. In collaboration with Oregon State University, construction at PacWave South began in June 2021. Other wave energy efforts in the region are ongoing, including a PacWave North development and the O.H. Hinsdale Wave Research Laboratory.
PacWave South Wave Energy Test Facility
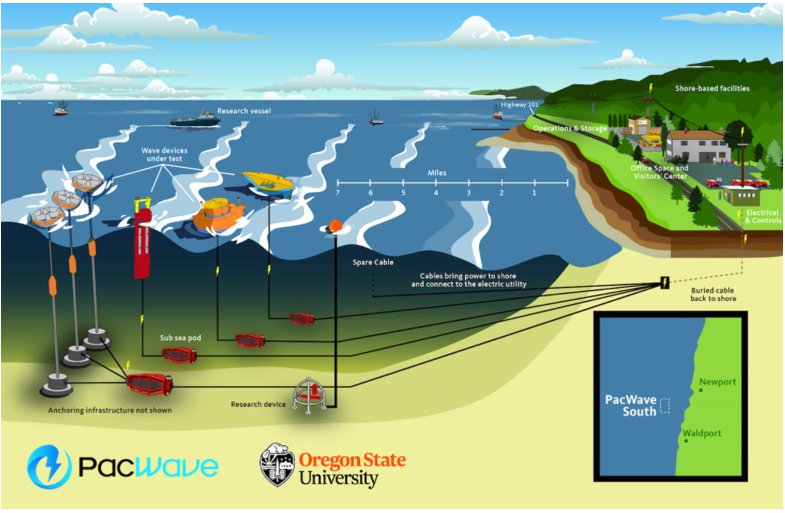
*Image from PacWave
Carbon Capture and Sequestration
Carbon dioxide (CO2) capture and sequestration (also sometimes referred to as storage or utilization) represents a group of technologies that aim to reduce or extinguish carbon dioxide emissions from the generation of electricity and either permanently store the carbon or reutilize it. There are traditional carbon capture technologies, which primarily follow the process outlined in the figure below. Regardless of the end-use, either injecting the carbon underground for storage or re-using the carbon for another purpose (like enhanced oil recovery), the reheating process of creating a pure CO2 stream requires additional load from the plant, reducing overall net plant output. There are only 22 carbon capture plants in operation globally, and only two are power plants (both coal) – the Petra Nova in Texas and Boundary Dam Power Station in Saskatchewan.
Traditional Carbon Capture in Power
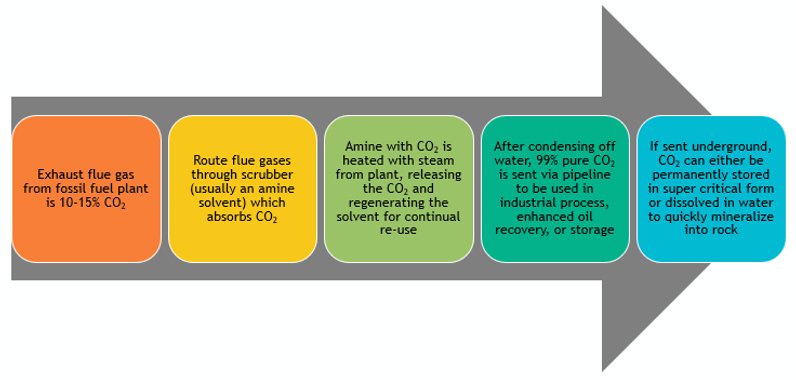
In addition to traditional carbon capture and sequestration technologies, there are emerging technologies and test projects that enhance and build on the original concepts. Some of these include the closed-loop CO2 using Allam-Fetvedt Cycle technology (e.g. the NET Power Plant,) coal gasification with carbon capture and sequestration (e.g. the Kemper Power plant in Mississippi), and accelerating the process of turning CO2 into rock underground (CarbFix in Iceland). While not all of these innovations have been successful, and some have been quite costly, there may be advances in the future that make these technologies applicable to the region.
Description of Proxy Emerging Technology Reference Plant – Small Modular Reactor
The Council decided to base the proxy emerging technology reference plant on small modular reactors – making clear that if and when it is selected in the models or the plan’s resource strategy, it should be assumed to be representative of any emerging technology.
The proxy emerging technology reference plant is based on the NuScale technology and the Utah Associated Municipal Power Systems (UAMPS) Carbon-Free Power Project (CFPP). (Since the reference plant was developed, the CFPP configuration has been modified, reducing the number of modules.) The reference plant consists of 12 nuclear reactor modules paired with 12 turbine generators, with a net capacity of 684 MW. This capacity is comparable to proposed offshore wind developments on the East coast. Each reactor is encased in its own containment vessel and housed below the ground, in a water-filled pool. The entire plant footprint, including storage for spent fuel, takes up about 35 acres – according to preliminary designs by NuScale. The capital cost estimate was developed through a combination of costs that included the much higher ‘first of a kind cost” and the anticipated cost reductions from a “nth of a kind project.” The anticipated fuel cost was incorporated into the fixed cost.
The reference plant and costs include all siting and licensing, infrastructure, step-up transformers, switchgear and interconnection facilities, and security, control, spent fuel management, waste disposal, and maintenance facilities. For modeling purposes, the earliest in operation date is 2030.
Proxy Emerging Tech Reference Plant – Small Modular Reactor: 2021 Power Plan Reference Plant
Reference Plant | Proxy Emerging Technology – Small Modular Reactor |
Technology Type | NuScale SMR technology |
Configuration | 12 modules x 12 turbines |
Nameplate Capacity (MW) | 684 MW |
Location | |
Avg. Annual Capacity Factor | – |
Round-trip Efficiency | – |
Heat Rate – HHV (Btu/kWh) | 11,000 |
Economic Life (Years) | 40 |
Tax Benefits | – |
Development Period (Yrs) | 4 |
Construction Period (Yrs) | 4 |
Earliest In-Operation Date | 2030 |
Overnight Capital Cost ($/kW) | $5,400/kW |
All-in Capital Cost (pre-tax benefits, 2030 operation) ($/kW) | $7,040/kW |
All-in Capital Cost (w/ tax benefits) ($/kW) | – |
Fixed O&M Cost ($/kW-yr) | $121.40/kW-yr |
Variable O&M Cost ($/MWh) | $1.63/MWh |
Transmission | BPA P2P Long-term Firm |
Deferred Transmission and Distribution ($/kW-yr) | – |
Maximum buildout (MW) | 3,420 MW (5 units) |
*All costs in 2016 year dollars. For more details and definitions of resource attributes, see Definitions of Reference Plant Components[GC4] .
Maximum buildout: The maximum potential for the emerging technology proxy plant is based on the realistic production and development of small modular reactors in the region during 2030 and 2042. After discussions with NuScale and the GRAC, the Council settled on 5 units, equating to a maximum of 3,420 megawatts capacity. Because this is representative of all emerging technologies, if the maximum buildout had been binding (if the models had selected all options in the timeframe) we could have modified this to be more reflective of other emerging technology alternatives that could be developed in the same timeframe.
Forward capital cost curves: A forward capital cost de-escalation rate of 0.05% annually has been applied to the emerging technology costs to reflect gradual price improvements. Since this is a proxy for all emerging technologies, it is expected variation in long term cost trends. By the end of the planning horizon in 2042, the emerging tech proxy reference plant capital, fixed O&M, and variable O&M costs are estimated to have improved (decreased) by 12%.
Emerging Technology Proxy (SMR) – Capital Cost (2016$/kW)
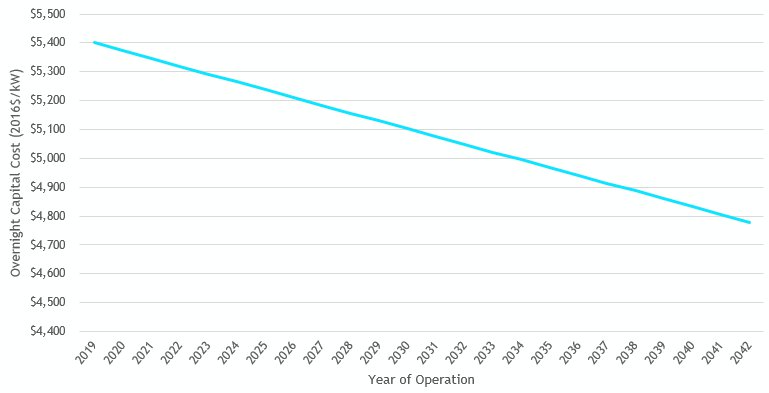
More information: For background analysis, see the initial presentation on the emerging technology reference plant from the May 2020 GRAC meeting. Please note that some of the material in the presentation may have been updated between that meeting and the draft plan. In addition, see MicroFin for supplementary inputs and analysis.